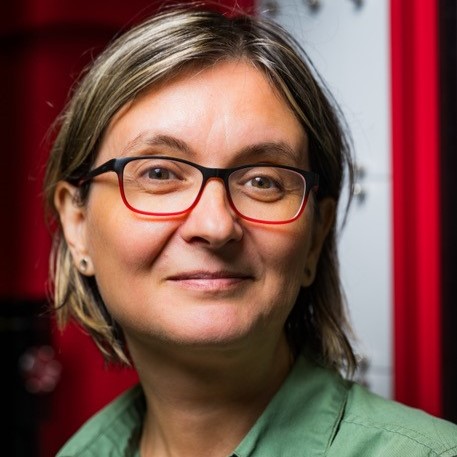
Correlated Topological Materials in a "New Light"
Emilia Morosan
Approved for Public Release
This research program is a basic science effort aimed at the discovery of new correlated topological systems. While Moore's law has successfully predicted the exponential growth of electronics for nearly 50 years, the optimization in size, cost, and power efficiency will plateau in 2025, as miniaturization nears the atomic limits. Evolving past the limitations of Moore’s law requires a paradigm shift in materials (“beyond silicon”), functionalities (electronic vs. optical responses), and control. We address this imperious need with a project based on new materials, new functionalities, and new control. Correlated topological systems hold significant potential for such a transformative leap, wielding the combined power of spin, charge, lattice, and topological degrees of freedom. This project will (a) discover new correlated topological materials that will enable continued miniaturization due to their distinct surface and bulk properties; (b) achieve new functionalities such as new device architectures with minimal heat dissipation and low power consumption, fault-tolerant computing with inherent robustness against decoherence, defects and environmental noise, high sensitivity for quantum sensing; (c) overcome the limitations of electronic transport by using light (instead of electrons) for new control with high precision by selectively exciting quantum states. The main objectives of this project are:
Objective 1. Discovery of quantum materials using a novel crystal growth technique: We will develop and build a new high temperature furnace for flux growth with in-situ decanting. This major and timely advance in crystal growth will allow us to reach previously-inaccessible material spaces (topological superconductors, 3D flat band intermetallics, quantum spin liquids, unpredicted materials and functionalities) where their expected sensitivity and tunability is commensurate with the high temperatures at which they form.
Objective 2. Use a precise design principle for novel Weyl-Kondo topological materials: The new furnace will enable the growth of new classes of materials, including Weyl-Kondo (WK) semimetals. These combine Kondo correlations (hybridization between core and conduction bands) and non-symmorphic crystal structure (fractional lattice translation with mirror or rotation symmetry). Weyl-Kondo semimetals are ideal platforms for new Berry phase engineering for quantum information processing, or for novel devices using the spin-locked chiral fermions.
Objective 3. Investigate the coexistence of real- and reciprocal-space topology by identifying linear band crossing in materials with real-space Berry phases and non-coplanar spin structures. The properties (nm size, stability, mobility) of these spin textures can be harnessed for highenergy data storage, concurrent topological and quantum Hall effects (THE, QHE), enabling read-out of spin-state information using THE, dissipation-less transmission using QAHE chiral edge states.
Objective 4. Light-tuning of quantum materials in a cavity allows for probing and dynamic manipulation of quantum states; deconvoluting surface and bulk properties inherentlydistinct in topological materials; inducing topological phase transitions with frequency control.
At the heart of the proposed research is the training of the next generation of scientists with expertise in materials design and crystal growth. Graduate students, postdoctoral researchers, and undergraduate students will be trained in state-of-the-art crystal growth techniques, materials characterization and instrument engineering. The research itself will rely on the synergy between theoretical insight, precise materials synthesis, structural, electronic and magnetic characterization.