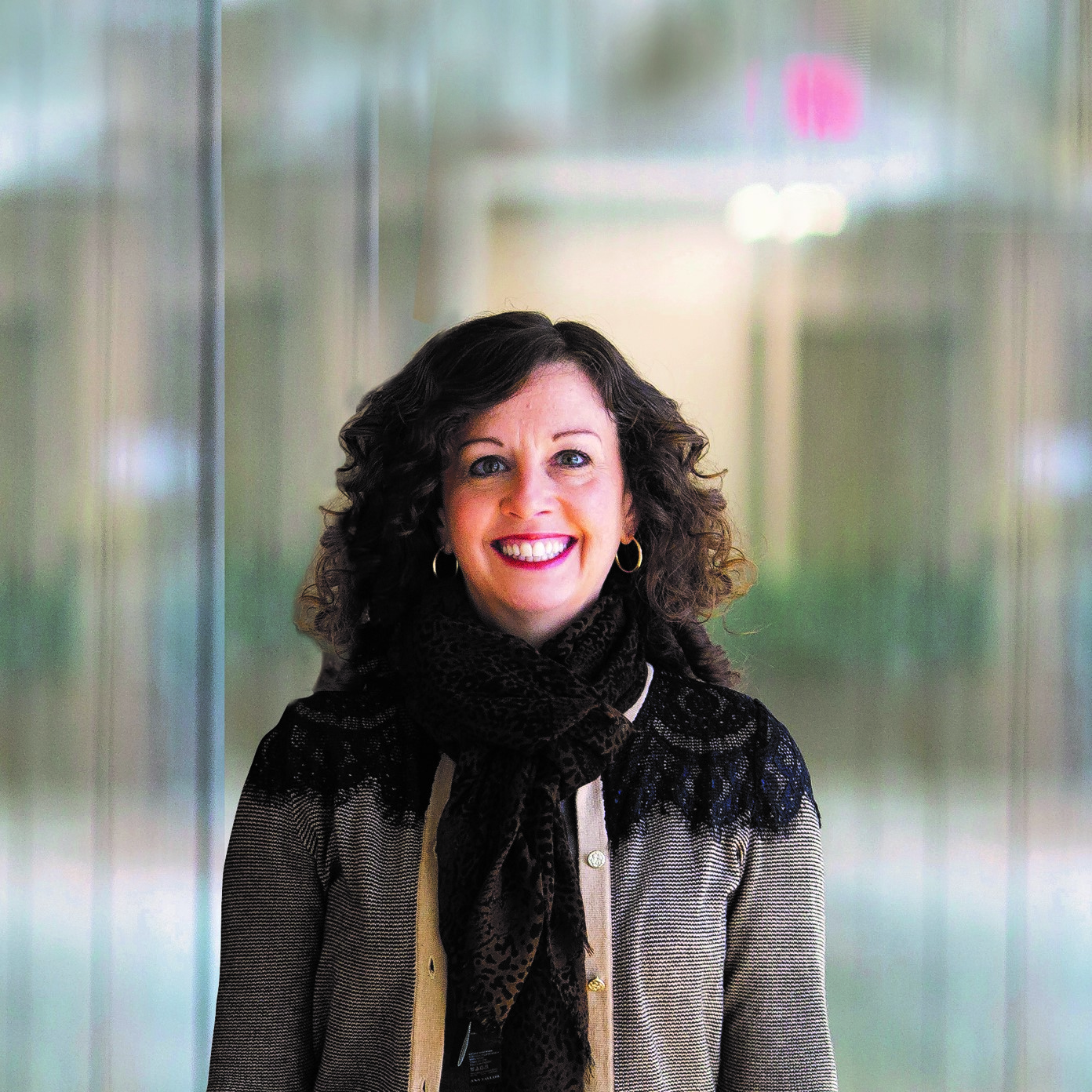
Abstract, 2022
(Approved for Public Release)
Nanoparticle self-assembly into colloidal crystals holds great promise for making materials with unprecedented combinations of properties. Partnership between experiment and simulation has yielded tremendous knowledge and insight over 20 years that is now used for design and synthesis. Yet no theory allowing for rapid, first principles prediction of structures from nanoparticle exists. Meanwhile, rigorous theory and software packages have been supporting atomic crystal design and structure prediction for decades. Remarkably, nanoparticles and atoms can assemble into identical structures, differing only on length, time and energy scales, suggesting that a parallel theory may be possible. Nanoparticles can even form these structures via entropic bonding – a new way of describing the entropic forces that drive ordering of particles due solely to their shape. Simple shapes self-assemble via entropic bonding into extremely complex structures also seen for atoms, with unit cells containing hundreds of particles. Pursuing the parallels between entropic and electronic bonding of nanoparticles and atoms, respectively, has the potential to transform nanoparticle design and synthesis and provide deep, foundational understanding of the relationship between valency and structure applying to all types of materials building blocks.
Our objective is to develop a foundational theory of entropic bonding based on statistical thermodynamics and use it to discover the fundamental connection between valence and structural complexity in matter. By mapping entropic bonding onto electronic bonding in hundreds of different crystals to relate shapes with atomic elements, we will investigate why and how atomic valence and colloidal particle shape can produce identical, complex crystal structures despite wholly different forces at play. By discovering relationships between valency and structure, we seek to expand the Periodic Table of Atomic Elements into a multi-dimensional Periodic Table of Shapes and develop predictive design rules for crystals of any building blocks. We will generalize entropic bonding theory using analytical approaches to include explicit nanoparticle interactions. We will impact DoD capabilities by making this generalized theory available through our open-source software and perform the first ab initio predictions of nanoparticle superlattice design. Finally, we aim to gain fundamental insight into entropic forces in biological cells, where the crowding of shapes is prevalent and entropic bonding is underappreciated. We will develop and test our ideas in a tight theory-modeling-simulation feedback loop, validating our work using simulation as well as experimental data from the literature and from collaborators. Anticipated outcomes include a transformational paradigm shift in our understanding of structural complexity, foundational new knowledge on the entropic bond, a new and powerful structure prediction method for colloidal crystals analogous to the electronic structure methods used by materials scientists for atomic and molecular structure prediction. Potential applications include self-assembled materials for novel photonic, plasmonic and mechanical properties, including applications of interest to DoD such as optical microsensing, broadband circular polarizers, quantum information platforms and more. Students and postdocs will be trained in nanoscience, computation, simulation, and applications. Foundationally, our project has the potential for transformative impact on science by demonstrating the novel, disruptive and paradigm-shifting notion of the entropic bond. If successful, our findings are of the type that will find their way into textbooks alongside the usual chemical bonds we all learn about in kindergarten
Abstract, 2009
Smart, Autonomous, Adaptive
Phenomena in Self Organizing,
Reconfigurable Materials