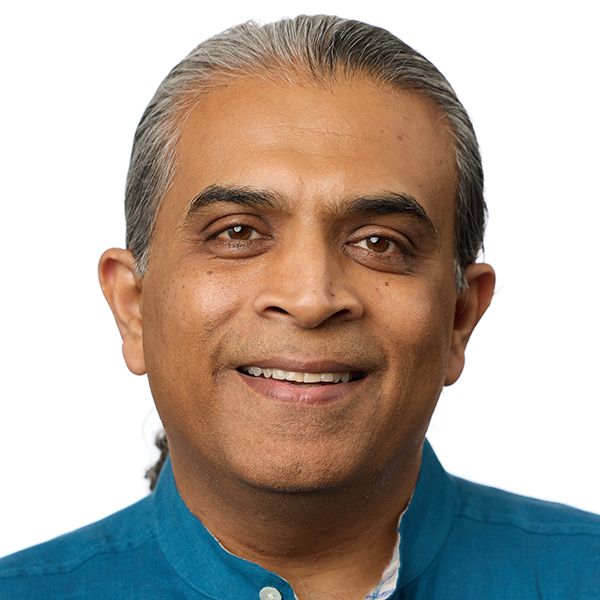
Siddharth Ramachandran, Boston University
Approved for Public Release
ABSTRACT
The overall goal of the proposed research program is to study light-matter interactions in the presence of angular momentum selection rules. The fundamental nature of these studies would impact applications as diverse as quantum networks, sensing, high-speed signal processing, imaging and manufacturing. The influence of the orbital angular momentum (OAM) on light’s coupling with matter is just beginning to be understood. For instance, opto-mechanical coupling of light through Brillouin interactions is known, but acoustic phonons carrying OAM, and hence the influence of this degree of freedom, has not been studied. Similarly, quadrupole transitions of electronic states in an atom have been achieved using excitation with light carrying OAM, but the complexity of such experiments implies that this field remains nascent. Finally, theoretical calculations posit significant advantages for implementing laser Wakefield acceleration of electrons to realize the dream of table-top energetic, coherent X-Ray sources, but implementation of these schemes remain unsuccessful.
Two common problems with investigating light-matter interactions with angular momentum states of light are: (a) the light beam is itself a higher order eigenstate, which suffers from modecoupling instabilities in the presence of cylindrical or spherical asymmetries in the optical system, or from perturbations such as turbulence; (b) OAM carried by these beams is maximized at the beam center which, due to its singular nature, is dark (i.e. carries no energy). This makes these more exotic transitions rather weak, and hence hard to observe, manipulate or exploit.
In this program, we leverage recent advances in the development of air-core optical fibers that provide unprecedented stability for a large ensemble of optical OAM modes – tests reveal the ability to conserve angular momentum of select light beams for up to kilometer lengths in suitably designed fibers (in contrast, in free-space, interaction lengths are limited to the Rayleigh focusing range of 0.1-1mm). The air-core that these fibers typically feature can also incorporate atoms, ions, molecules or electrons that need to be excited, trapped or accelerated, as the need might be. Finally, such optical fibers can be designed to support fractional, and not just integer, OAM states, in which the spin and orbital angular momentum of the photon are not separable. Hence, a suitably designed angular-momentum mode supporting air core optical fiber represents an ideal platform with which to study light matter interactions and probe obscure transitions that are forbidden for conventional light due to angular momentum selection rules.
Our study will have four distinct thrusts. Two thrusts comprise studies of light interactions with condensed matter: (1) acoustic phonons coupled via opto-mechanical interactions, mediated by angular-momentum selection rules; and (2) optical phonons coupled via Raman scattering, controlled by spin-orbit coupling that governs the propagation of light pulses. The other two thrusts involve (1) studying quadrupole electronic transitions in atoms and molecules, and (2) studying electron acceleration using OAM modes of the fiber. Using these unique tools, we expect to probe fundamental effects related to quantum theory, while also developing applications for classical and quantum optical communications, signal processing, sensing and high-power lasers.