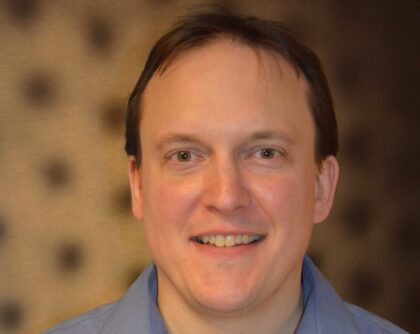
MARTIN ZWIERLEIN, MIT
Approved for Public Release
ABSTRACT
Complete coherent control over a collection of quantum systems and the ability to entangle arbitrary pairs of them are core requirements for digital quantum computation. After decades of work, this goal has been achieved so far only for about a dozen particles in traps, while factorizing useful numbers would require myriads of qubits. Recent years have shown vast progress on analog quantum computation, where a many-body Hamiltonian is realized with ultracold atoms. An important class of problems where classical computation fails involves strongly interacting fermions. Examples are found in all of chemistry, where many electrons form the Coulomb gas, in condensed matter physics with its grand challenge of understanding high-temperature superconductors, in nuclear physics in modelling nuclear fission, and in astrophysics with questions on the equation of state, dynamics and collisions of neutron stars. Limitations in the current approach with neutral atoms are the speed and the contact nature of interactions, which severely restrict the class of problems that can be simulated.
Here I propose a versatile and high-speed analog quantum computer using ultracold fermionic molecules, able to solve a large variety of complex many-fermion problems and to elucidate the emergence of many-body entanglement and its role in defining material properties. We will place the molecules in a two-dimensional array under a quantum gas microscope, allowing for singlemolecule detection with high fidelity. The strong long-range dipolar interactions allow simulating a wide range of Hamiltonians. We will in particular realize extended Fermi-Hubbard models with next-neighbor interactions and density-dependent tunneling, relevant for understanding high-temperature superconductivity. We will create an entirely new state of matter enabled by dipolar interactions, a topological superfluid of fermionic molecules in onedimensional arrays, and via local spectroscopy detect the presence of Majorana bound states. Furthermore, given the second-long coherence times of ultracold molecules demonstrated at MIT, we will turn our platform into a quantum storage device. Employing dipolar interactions, we will build effective spin models with thousand times faster spin-exchange rates compared to neutral atoms, and without the use of unstable states as in Rydberg chains. We will demonstrate quantum gate operations with chosen pairs of molecules and thus explore digital quantum computation with arrays of ultracold molecules.
The work will have potentially disruptive impact on DoD capabilities in quantum information storage and processing, and in providing new avenues towards creating novel materials for lightweight motors and data storage, through quantum simulation of electronic systems that are impossible to solve on classical computers. In particular, its outcomes will inform current theoretical efforts to solve the Fermi-Hubbard model and to provide the design principles for superconductors with high critical temperature. It will enable the creation of a novel state of matter hosting topologically protected states that allow robust encoding of quantum information.
The research will establish ultracold molecules as a competitive platform for digital quantum computation, with advantages over all current efforts in solid state qubits, ions, and neutral Rydberg atom arrays. The research will educate and train students and postdocs for the defense workforce in techniques ranging from ultrahigh vacuum, optics and lasers, electronics, computer control, quantum mechanics of atoms and molecules to the control of many-body quantum physics.