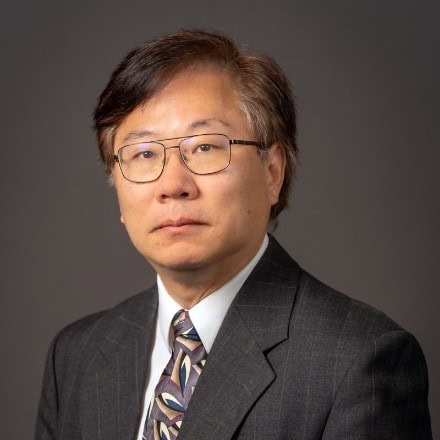
Project Abstract/Summary
The objective of the proposed research is to develop innovative, interfacial “phase” diagrams. The development of bulk phase diagrams and CALPHAD (calculation of phase diagrams) methods established the foundation for modern materials science, and interfacial phase diagrams (also called complexion diagrams) and their construction methods developed in the proposed project can have equally significant impacts.
Bulk CALPHAD methods will be extended to model grain boundaries (GBs) and free surfaces. Experimental validation will be accomplished using advanced electron microscopy and measurement of interfacial transport properties. First-principles density functional theory (DFT) calculations will be conducted to support thermodynamic modeling and to forecast GB cohesion/embrittlement. Moreover, ab initio and classical molecular dynamics (MD) will be carried out to forecast interfacial kinetics for use in predicting useful trends in sintering, grain growth, Coble creep, and ionic mobility. Using Mo-based alloys and Y2O3-stabilized ZrO2 (YSZ) as the (initial) model metallic and ceramic systems, respectively, computed GB diagrams will be used to forecast and control the fabrication and mechanical properties of advanced structural materials. An additional, transformative concept is to utilize 2-D interfacial phases to achieve superior properties unattainable by conventional bulk phases; battery materials will be used to demonstrate broader technological impacts (beyond structural materials).
Interfacial phase diagrams and the methods to compute and use them – the key outputs of this research – will enable entirely new ways to tailor the processing and properties of many engineered materials for defense applications. Specifically, GB diagrams can be used for 1) optimizing fabrication pathways to utilize desired interfacial structures during processing to control microstructural evolution; 2) designing heat treatment recipes to adjust interfacial structures to improve mechanical or physical properties; and 3) forecasting interface-controlled, high-temperature material properties. In addition, analogous surface diagrams can be used to design spontaneously-formed “dream coatings” with self-regulating nanometer thicknesses.