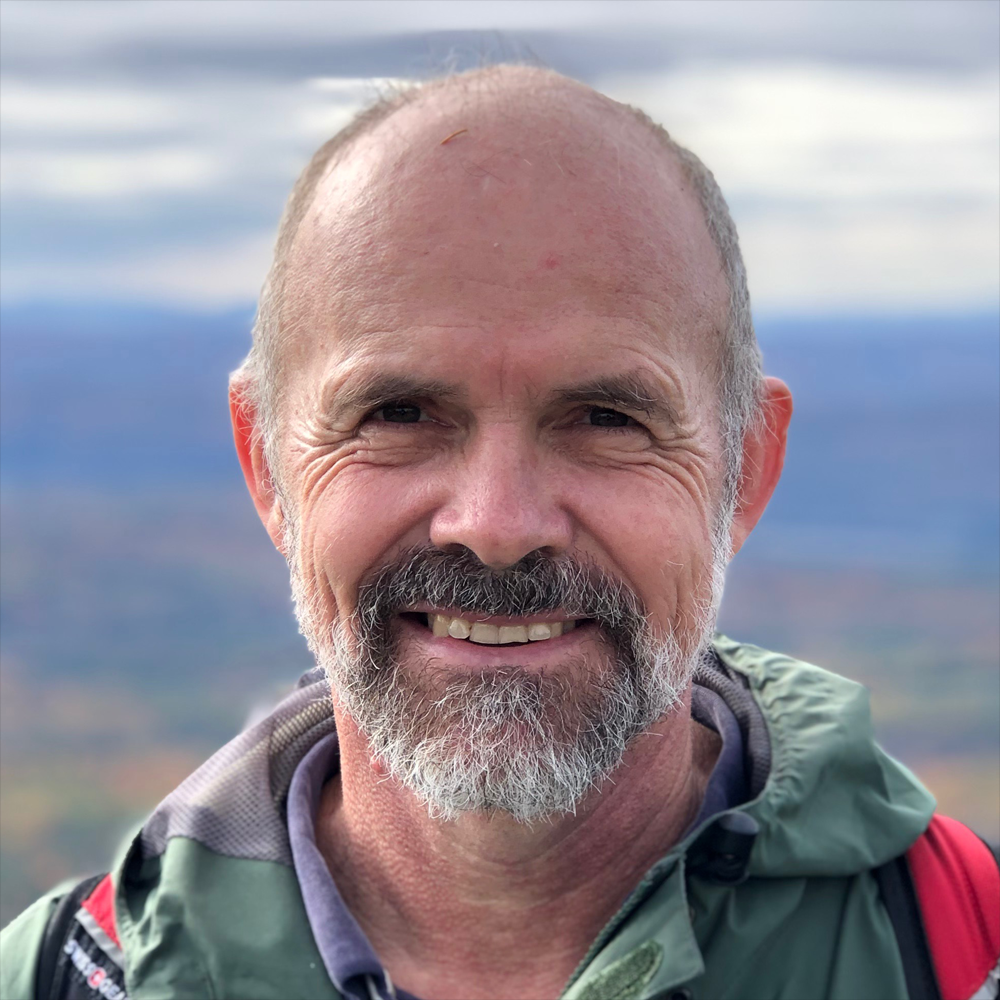
Building a Phonon-Based Quantum Computer
Andrew Cleland
Approved for Public Release
My research group has piloted the integration of high-fidelity superconducting qubits with surface acoustic wave (SAW) devices. Using this new technology, we have demonstrated the on-demand generation and detection of individual microwave-frequency surface acoustic wave phonons. We controllably emit individual phonons from excited-state qubits, sculpting their time-symmetric waveforms, directing each phonon towards a receiving qubit. We can generate qubit-phonon entanglement of the form (| 0⟩ + | 1⟩)⁄√2, when captured yielding phonon-mediated qubit Bell states (| ⟩ + | ⟩)⁄√2. We have developed an acoustic beamsplitter, which splits a single phonon input |1⟩ into a Bell state phonon output (|0⟩ + |1⟩)⁄√2, in turn yielding a qubit Bell state. By interfering two input phonons at the beamsplitter we have demonstrated the acoustic HongOu-Mandel (HOM) effect. The optical HOM effect notably forms the key element for gates in linear optical quantum computing; here we envision similar possibilities using mobile phonons.
We propose to significantly extend and expand this technology by developing measurement-based quantum computing as well as gate-based quantum computing, using phonons as mobile qubits. Measurement-based quantum computing affords lower fidelity requirements for a number of quantum applications, and is particularly suited to mobile qubits for which qubit gates are challenging to implement. Pending other research in our group on developing phonon phase gates, we will also explore circuit-based quantum computing. The main thrust of our research effort will be to: (1) Extend our dual-port, single-rail beamsplitter to a four-port dual-rail beamsplitter, that will allow fan-out and generation of dual-rail entangled phonons; (2) develop a phonon “click” detector that announces the arrival of a phonon, analogous to the ubiquitous photonic “click” detector; (3) develop the means to generate phonon cluster states; and (4) integrate these new capabilities for preliminary demonstrations of mechanical quantum computing. This computer would be tightly integrated with, and measured by, a set of superconducting qubits, generating and manipulating phononic cluster states. In addition to this main effort, pending other research, we will explore Lamb wave-based quantum acoustics, using suspended membranes or acoustic racetracks, which promises lower loss than our current bulk surface acoustic wave technology.
This project focuses on developing a new quantum computing architecture that melds quantum circuits-based superconducting qubits with mobile phononic qubits. The architecture is entirely solid-state with straightforward transduction between the two qubit families. The use of phonons in this architecture promises extensions to other exciting possibilities: Optomechanical up-conversion to photonic encodings for distributed computing; hybrid quantum systems allowing coupling of the phonons to e.g. silicon-vacancy centers in diamond, which in turn can access nuclear memories; coupling to rare earth ions, with long lifetimes and affording a coherent optical interface; and coupling to mechanical resonators in turn coupled to cold atoms.
A solid-state, measurement-based quantum computer could have important implications for Department of Defense priorities, providing a clear and compelling means for implementing NISQ-level quantum computing algorithms with easier requirements for fault-tolerance as well as much better connectivity, increasing the likelihood for the successful implementation of quantum algorithms related to information security and quantum simulations with defense applications.